Water Pumps
FAMA BUYER’S GUIDE TC024 WATER PUMPS
WATER PUMPS
Prepared by the FAMA Pump Subcommittee
This guide does not endorse any manufacturer or product
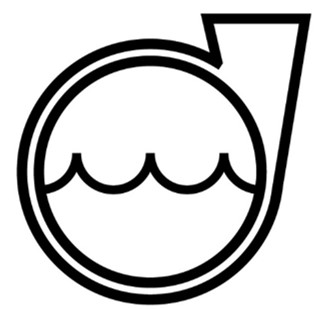
Contents
Introduction
This guide is intended to provide fire service personnel with information about the various types of pumps, their capacities, mounting locations on fire apparatus, drive systems, control and monitoring systems, and valve types that are available for fire apparatus.
As with any engineered system design, there are pros and cons to each configuration and not all are represented here. It is the responsibility of the purchaser to determine what best meets their needs and to specify this information to prospective apparatus bidders, sellers, and manufacturers.
As technology is continually evolving, this guide is presented as a general information and educational piece. The various options and auxiliary equipment presented may not be available from every manufacturer, and some may be mutually exclusive.
For more information, contact the FAMA Member pump, equipment, and apparatus manufacturers listed at www.fama.org.
The National Fire Protection Association Standards define voluntary minimum standards for automotive fire apparatus. The two most widely used Standards are:
NFPA 1901®, Standard for Automotive Fire Apparatus
NFPA 1906®, Standard for Wildland Fire Apparatus
The current editions should be referenced when specifying new apparatus.
Within these Standards, pumps are divided into three main headings each with specific minimum performance criteria:
- Fire Pump,
- Auxiliary Pump, and
- Ultra-High Pressure (UHP) Pump.
In Canada, ULC S515 Standard for Automobile Fire Fighting Apparatus is the accepted standard to reference.
Overview
Modern fire apparatus pump systems are comprised of many components utilized to move and control water from a source. They use power to create or increase pressure to move the water through piping and valves, then discharge the water to hose lines or other appliances to be directed to another location, applied to a fire, or other desired target.
This guide will present in general terms:
- Pump Types
- Drive Methods
- Pump Locations
- Pump Capacity Ratings
- Intake Plumbing
- Discharge Plumbing
- Governor Types
Pump Types
Centrifugal
Centrifugal pump design is the most typical for Fire Pumps and Auxiliary Pumps in modern fire apparatus and its proven design has been used in fire apparatus for well over a century. In a basic centrifugal pump, typically a single casing, water enters through the pump inlet into the center (eye) of the impeller which is spinning on a shaft. Water is spun outwardly through the impeller vanes and into a volute casing resulting in increased pressure. The water is then discharged at a higher pressure than when it entered the pump inlet. Centrifugal pumps have the ability to draft (draw) water from an unpressurized water source with the aid of a primer. Centrifugal pumps are available as single stage or multi-stage: such as two-stage and three-stage designs. Stages refer to the number of impeller(s) and water path(s) within the centrifugal pump.
Single Stage
In a basic Single Stage Pump, water enters through the pump inlet and into a single spinning impeller. Water then moves outward through the impeller vanes and into a volute casing. Pressure rises in the casing resulting in a higher pressure out the pump discharge. A Single Stage Pump will not have a Transfer Valve.
Multi-Stage
In a Multi Stage Pump, there are two or more impellers that work together to provide the pump’s required pressure and flow. Most designs have the impellers mounted on a single shaft, though, occasionally, they may be separate. (See “booster” pumps below)
Multi-stage pumps are most typically used to develop high discharge pressures from 250 psi to 1500 psi. Each impeller adds to the pressure it receives from the stage before it, typically providing output pressures significantly higher than that of a single stage pump.
Many multi-stage pumps, especially two-stage pumps, will be equipped with a transfer valve. The transfer valve allows the different stages of the pump to operate in either a pressure or volume mode. In the pressure mode, the stages are linked in series so that the output of the previous stage is routed directly to the subsequent stages, compounding the pressure as described above.
In the volume mode, the stages are run in parallel so that the flow from each stage is basically added together. Higher flows can be achieved.
Some pumps may be equipped with a high-pressure “booster” pump. The booster pump impeller will likely have its own casing separate from the casing for the other stages, though it may still be driven off a common gearbox. This pump may be plumbed in series with the other stage(s) for high pressure flow, or it may be plumbed as a stand-alone pump allowing simultaneous high and low pressure flow. A transfer case may be provided to switch between the modes.
Cast Construction
A single or two stage pump of cast construction typically has impeller inlet(s) and waterway(s) cast into one complete cast pump casing. The transfer valve on a two stage pump is located in an area of the pump body where water can be controlled to either flow from one stage to another (pressure mode) or directly from each stage out the discharges (volume mode).Single stage and two stage pumps are available with iron or bronze castings.
Fabricated Construction
A single or two stage pump of fabricated construction will typically have a separate pump casing from the inlet and discharge piping. In the two stage pump, piping is used to connect one pump stage to another and the transfer valve is in a location within the fabricated piping so water can be controlled to either flow from one stage to another (pressure mode) or directly from each stage out the discharge(s) (volume mode). Pump and apparatus manufacturers have the capability of providing stainless steel intake and discharge plumbing / piping on certain pumps.
Positive Displacement Pumps
Positive Displacement Pumps are most often used for Ultra High Pressure pump applications. A positive displacement pump is different from a centrifugal pump in that these pumps operate by drawing water into a chamber then “squeezing” it out under pressure. Examples of positive displacement pumps used in the fire service are piston pumps, rotary vane pumps, and gear pumps. Each time the pump spins, a specific amount of water is moved. Because of this action, positive displacement pumps are capable of delivering constant flow at very high pressures at a constant speed.
Positive displacement pumps are restricted to low volumes (typically <50 gpm) due to size and complexity. They are most commonly used for ultra-high pressure pumping systems that develop pressures well in excess of 1,000 psi, for foam injection systems, or for priming the main centrifugal pump.
Water supplied to a hose by a positive displacement pump will behave differently than a line supplied from a centrifugal pump. For centrifugal pumps, reducing the backpressure (i.e. opening a valve from half open to fully open) will result in a noticeable increase in flow (up to the capacity of the pump). However, for a given pump speed, a positive displacement pump will maintain constant flow, and it will do so regardless of the backpressure on the system. As an example, if the flow rate is 18 gpm at 1100 psi at the nozzle, the flow rate will remain about 18 gpm even after the nozzle is removed. Positive displacement pumps are also likely to cause pulsations in the discharge line.
Drive Methods
The drive method is how the pump gets its power to turn the pump shaft thus creating water flow and providing pressure.
MIDSHIP MOUNTED TRANSFER CASE DRIVE
Overview:
Midship split driveline pumps are typically located in the middle of the apparatus, between the transmission and rear drive axle as part of the apparatus drive line. The pump and gear box are most commonly mounted together as a single unit, and the pump is driven directly from the gear box, by means of a chain, gears, or belt. A different configuration of a split driveline pump is to have the gear box located in the driveline, and the pump mounted in the rear of the vehicle. The pump is driven by means of a power shaft, similar to a power take off shaft, instead of a chain or a belt.
There are two, or more, drive shafts, connecting the engine transmission to the pump gearbox and the pump gear box to the rear drive axle.
A control must be installed inside the driver’s compartment to select between “Road Mode” and “Pump Mode.” When “Road Mode” is selected, power from the engine and transmission is transmitted through the driveshafts, pump gear box and to the rear drive axle which propels the vehicle. The pump is not engaged when in “Road Mode.” When “Pump Mode” is selected, the power from the engine and transmission is transmitted through the driveshaft to the pump gear box to drive the pump. The rear driveshaft is not engaged and the vehicle will stay stationary.
Consideration must be given to the ratings of the pump, engine horsepower and RPM requirements.
The pump power curve, available from the manufacture, will determine certain requirements of other components. To ensure a reliable system, all components must work in conjunction with one another. It is important to note driveline design is a critical aspect of a transfer split case pump installation. Manufacturers of driveline components and, pump manufacturers should be consulted before any driveline design is implemented on a fire apparatus to ensure the integrity and reliability of the installation.
Typical Pump Sizes:
Pump sizes are determined by their flow capacity in Gallons per Minute (gpm). Common size ratings of Midship Split Driveline pumps available include:500, 750, 1000, 1250, 1500, 1750, 2000, 2250, 2500, 2750, 3000, 3500, 4000, 5000, & 6000 gpm.
Note: Pumps with a size of 3000 gpm or more are often referred to as industrial pumps.
Pro’s:
- Provides higher flow ranges, up to 6000 gpm, utilizing the power train to provide required power.
- Engine speed remains relatively constant to reduce fluctuations in pump discharge pressures.
Con’s:
- No pump and roll capabilities.
- Pump gear box is part of the driveline, making some repairs more difficult.
- If a problem occurs with a pump gear box, it may impede the vehicle from being driven.
- Typically more expensive than other types of pump drives.
- More difficult to install than other types of pump drives.
- More space is required.
POWER TAKE OFF (PTO) DRIVE
Overview:
There are two types of PTO drives. One receives power input from the main engine or transmission. The other indirectly supplies power to the pump through a hydraulic pump. For a PTO driven by the main engine or transmission, an appropriate PTO drive unit is mounted to one of the main engine’s or transmission’s PTO ports. A drive shaft is typically used to connect the PTO to the pump. Consideration must be given to the ratings of the PTO port, PTO drive unit, duty cycle, pump horsepower & RPM requirements. Inappropriate sizing of the PTO may lead to failures of the PTO drive unit.
Some applications will utilize a PTO driven hydraulic pump that in turn drives a hydraulic motor to turn the pump shaft. A control, typically located inside the driver’s compartment, is used to engage and disengage the PTO gear box.
The pump power curve, available from the manufacture, will determine some of the requirements of the other components. Designing a reliable system requires all components work in conjunction with each other. It is important to note the PTO is often considered the weakest link in a PTO drive system. The PTO for the pump design should be verified with PTO suppliers as well as pump manufacturers to ensure the reliability and integrity of the pump drive system.
Sizes available:
250, 350, 450, 500, 750, 1000, 1250, & 1500 gpm are the most common size ratings for the use in a PTO Drive system.
Locations:
PTO driven pumps can be located in many positions on a fire apparatus. Typical locations are: front mount pump; midship (non-split driveline) pump; rear mount pump.
Pro’s:
- A PTO driven pump typically allows for pump and roll (pump & move) where the vehicle can be driven using the vehicle’s normal chassis engine, transmission and drive train to propel the vehicle, while the PTO powers the pump so that water, foam and/or Compressed Air Foam System (CAFS) can be discharged while the vehicle is in motion.
- Unlike a split shaft pump gearbox configuration, PTO driven pumps and drive components are not integral with the drive train components between the transmission and drive axle(s).
- If a problem occurs with a PTO pump gear box, it is unlikely that this will impede the vehicle from being driven.
- Typically less expensive than other types of pump drives.
- Easier to install in most applications.
- Less space is required.
Con’s:
- For PTO’s mounted to the main engine or transmission, during pump and roll operations, pump pressure can vary greatly due to throttle position of apparatus, possibly causing variation in water discharge pressure. A hydraulically driven PTO pump may reduce such variation as the hydraulic pressure can be governed to maintain a more consistent water discharge pressure.
- For PTO’s mounted to the main engine during pump & roll the chassis engine may require a higher RPM to maintain proper pump discharge pressure. This may be too high an RPM level for the speed desired to drive the vehicle. If the vehicle’s brakes are utilized to maintain the desired speed, over-heating and increased wear on the brake components may occur. A hydraulically driven PTO pump may reduce or eliminate such issues.
- Flows are typically limited to a maximum of about 1500 GPM.
- Duty cycles of PTO components may increase service and maintenance requirements, and may decrease component life.
AUXILIARY ENGINE DRIVEN (DIRECT DRIVE)
Overview:
Auxiliary engine driven pumps are coupled directly to their own engine which is separate from the main engine. Auxiliary engine driven pumps are often used for Auxiliary Pumps. In cases where the pump is rated less than 500 gpm, the pump may be directly connected to the engine output shaft so the pump is spinning every time the engine is running. Some manufacturers will use a gear box between the engine and pump that either increases or decreases the pump speed to engine speed. Some pumps may be equipped with a clutch to disengage the pump from the engine.
Sizes available:
Most auxiliary engine driven pumps will be low horsepower pumps designed to flow relatively low volumes (typically <500 GPM). Gasoline engines are available to drive pumps up to about 200 gpm at 100 to 150 psi for mid-range pumps; 100 gpm at 100 to 250 psi and 50 gpm at 300 to 400 psi for high pressure pumps. Small diesel engines are available to drive pumps within the same range as the gasoline units, however, the higher horsepower offered by the diesel engines will typically allow the diesel units to provide greater flows in the same pressure ranges. These pumps are used primarily for brush trucks or wildland/urban interface units built to meet the design practices identified in NFPA® 1906 – Standard for Wildland Fire Apparatus.
Larger capacity pumps up to 6000 gpm are also available, and are often used in industrial applications. Keep in mind that as the flow increases, the power required to drive the pump increases exponentially. Therefore, the size of the engine and the cost of the system will also increase exponentially. For that reason, large engine driven water pumps are often mounted on trailers or deployable pods for stand-alone specialty pumping systems.
Please see the pump capacity section for discussion on pressure.
Locations:
Auxiliary engine driven pumps are usually mounted in the rear of the apparatus behind the water tank. Occasionally, the pumps are cross-mounted behind the cab or in the apparatus dunnage area, either as a stand-alone pump module, or as an auxiliary pump to a chassis mounted PTO or split-shaft driven pump system.
Pro’s:
- Pump system is truly independent of the main engine allowing pump flow and pressure to be independently regulated without impact to systems driven by the main engine.
- Due to the independent nature of this system, it provides excellent pump and roll capabilities.
- If a problem occurs to an auxiliary engine and pump, it is unlikely that it will impede the vehicle from being driven.
- Often easier to service due to ease of access.
- Pump may have multiple control panels for in-cab and pump side operation.
- Remote mount control panels allow the pump controls to be located almost anywhere on the fire apparatus providing an optimum location for the pump operator to oversee the fire scene as well as keeping him safe from discharge lines and equipment.
Con’s:
- An auxiliary engine driven pump may be more expensive than other types of pump drives.
- When an auxiliary engine is used, useable space and payload capacity may decrease.
- Increased maintenance costs may be incurred due to the separate engine.
Pump Locations
Depending on pump drive type, there are various locations where the pump can be mounted. For split shaft/transfer case and PTO units the pump can be mounted in the:
- Front of the apparatus, ahead of the engine and front axle;
- Between the front and first rear axle, (Midship)
- Rear of the apparatus, behind the first rear axle.
With auxiliary engine driven pumps, pump locations can be behind the cab, in the panel can be located on top, on the side, and/or at the rear. The location doesn’t necessarily need to be near the actual pump. It is well advised to keep intake and discharge hose connections away from the pump operator for improved safety, reducing the risks associated with pressurized hose lines and tripping hazards. Each location has its advantages and disadvantages, depending on the department’s needs.
Pump Capacity Ratings
The size of the pump that is to be mounted on an apparatus is one of the most important decisions when developing the specifications for a new pumping apparatus. Often purchasers will buy an apparatus similar to what they’ve purchased in the past. That’s not a bad practice assuming the performance and operation of their existing fleet is adequate for the present and future needs.
Experienced purchasers believe most municipal pumper fire apparatus should be equipped with a pump that is rated at 1500 gpm or larger. That may be a good rule of thumb, however, to maximize the value of the apparatus you may wish to consider the following. Click here for pdf.
Pump Intakes
Any external connection that can be used to supply water to the pump is considered a pump intake. Typically, a fire pump will include a minimum of two (2) intake connections: one (1) larger diameter main inlet (sometimes called a “steamer”) connection and one (1) smaller auxiliary connection.
The “steamer” inlet size, and quantity, should be selected to supply the pump’s full rated capacity from draft. NFPA® 1901 Standard for Automotive Fire Apparatus contains a table that lists the maximum size and quantity of suction lines that may be used for testing of the pump from draft. Due to the necessity to supply the pump from draft, the main inlet connection will be positioned as close to the pump as possible with the shortest, straightest path possible into the pump impeller.
Auxiliary suctions are usually smaller connections used to feed the pump from a pressurized source such as a hydrant. Most apparatus equipped with the auxiliary suction will be equipped with a 2.5” connection. Auxiliary suctions must also be equipped with a valve.
It is not unusual for an apparatus to be equipped with additional inlet connections based on the size of the apparatus. For example, certain apparatus may include additional connections at the front and/or rear to maximize the flexibility of the apparatus to be supplied with water while the apparatus is positioned in the nominal location relative to the water supply. The key to remember is, the greater the distance between the hose connection and pump impeller, the more pipe, elbows, and other potential restrictions will be required in the plumbing. As a result, the draft capabilities of these additional inlets may be significantly less than the main inlet. On a typical pumper apparatus, it is not unusual for a front suction to have a draft capability of 70% or less compared to the main inlet. Reducing the restriction(s) to improve the flow may not be feasible if the route of the plumbing is not conducive for larger or straighter pipe runs.
INTAKE VALVE TYPES
It is good practice for the main inlet (or any inlet) to be equipped with an intake valve. The valve will allow the operator to set up to supply the pump from an external water source while still being supplied from the on board tank. When shifting between water sources, one valve can be opened while another can be closed.
In general, there are two choices for the types of valves to be used, internal or external. An internal valve is an integral component of the pump intake plumbing and typically a “butterfly” type valve. An external valve is mounted outside the pump and pump panel. It can be either a ball, butterfly, or piston type valve. Whether internal or external, these valves perform the same function in controlling incoming water flow to the pump through one or more of the pump’s primary water intakes. Both types of valves must have a means to expel air from the hose line when first charged. This prevents a slug of air from moving through the pump and hose lines to reach the firefighters at the nozzle. Either valve should also incorporate an automatic pressure relief feature to prevent unwanted pressure surges in the supply hose over pressurizing the pump and discharge hose lines.
Internal (integral) Intake Valves
Pros:
- Most can be controlled remotely from the pump operators position
- Operates from a draft or pressurized water source
- Protected from outside damage
- Does not increase the width of the apparatus
- Incorporates a manual override feature
- Provided by the pump manufacturer
Cons:
- More expensive than an external valve
- Butterfly design partially blocks waterway
- When repairs are needed, the apparatus, or pump, may need to be taken out of service
- May not be easily accessible or serviceable
External Valves
Pros:
- Some can be controlled remotely from the pump operators position
- Less expensive than an internal valve
- Most will operate from either a draft or pressurized water source
- Can be easily replaced or repaired without the pump or apparatus being out of service
- Incorporates a manual override feature
Cons:
- May not fit inside running boards, increasing the over-all width of the apparatus.
- More prone to damage
- Different manufacturer than the pump manufacturer
It is up to the purchaser to determine what is best for their operations, repair capabilities, and budget.
Pump Discharges
Discharges are any outlets on the apparatus that provide water flow under pressure from the pump. Most discharges are classified by the size of the hose connection at the exterior of the apparatus. Many departments may assume the valve and pipe sizes match the connection size. While this may be true, there are exceptions. Therefore departments should make it clear if they are referencing pipe or valve sizes rather than connection size. Discharges range in size from 1” to 8”, though common discharge sizes used on apparatus in North America are 1.5”, 2.5”, 3”, 4”, 5” and 6”. The size and location of the discharges are almost always selected based upon the anticipated use of the apparatus (e.g. where hoses or master stream devices need to be connected on the truck while on the fireground, including those lines that are going to be preconnected to the discharge).
Applicable standards from the NFPA have charts that identify the ratings of various discharges based on the connection size. The sum of all the discharge ratings on the apparatus should equal, or exceed, the total pump capacity ratings. Only discharges larger than 2.5” count towards the sum. The actual capability of a given discharge usually exceeds its ratings. The actual flow of a given discharge will be determined by the valve size, the plumbing size, restrictions in the plumbing (such as flowmeters), and of course the hose and nozzle size connected to the discharge.
Ratings for discharges as identified by the applicable NFPA standards are:
2.5” = 250 gpm
3.0” = 375 gpm
4.0” = 625 gpm
5.0” = 1,000 gpm
6.0 = 1,440 gpm
No discharge larger than 2.5” is allowed on the operators’ pump panel. The flows listed for each outlet size are minimum and are for rating purposes only. If piping and valving are sufficient, much higher flows for a given outlet size might be achievable.
PLUMBING SIZE
One of the most important factors that determines the flow rate from a discharge is the plumbing size which includes the valve size. Most departments will specify that the plumbing size should be equal to, or greater than, the discharge connection size to reduce friction loss in the piping. However, consulting with the manufacturer to ensure this does not create any problems is the recommended approach. It is not unusual for smaller plumbing size(s) to be required to clear obstructions on the apparatus, particularly when the discharge is located a large distance from the pump (such as at the front bumper). Additionally, it is not unusual for a smaller pipe size to be able to flow the expected rating of the discharge, particularly if the pipe run is short and relatively straight.
Note: Some departments may attempt to define a maximum pressure loss in the discharge piping. This may not be useful given the many variables to consider. Most manufacturers will design their plumbing system to supply at least 150 psi pump pressure. When a higher flow rate is desired, the specifications for the apparatus should state “The discharge must be capable of supplying X gpm at Y psi at Z engine speed.”
VALVE CONSTRUCTION AND CONTROLS
Full flow ball valves (ball valves) are the most commonly used on fire apparatus. A ball valve is manufactured with a ball which has a port machined through the center. When the ball turns one way, it blocks flow. When turned approximately 90 degrees, the port aligns with the pipe and allows flow. Because the diameter of the port is approximately equal to the inside diameter of the valve pipe connections and there are no other restrictions in the flow path (such as a valve stem) there is minimal friction loss.
These valve types usually require minimal maintenance such as replacing seals due to wear from normal use and do not require any lubrication. Locating the valves where they are easily accessible should be a consideration to allow for easy maintenance. Many of these valve types have what is called a “swing-out” design in which the valve body can rotate away from the flanges connecting it to the pipe so that seals may be accessed without having to disassemble much of the plumbing.
Valve Control Styles
Valve controls are available in three styles:
- Manual: These may be push-pull (e.g. T-handle), crank/handwheel (rotational), or swing. The common factor is a physical linkage between the operator’s control panel and the valve itself. These are the least expensive controls available and are the most commonly used. The main drawback to manual controls is the valves must be located relative to the control so that the geometry of the linkage is effective (e.g. there is no binding during valve operation) and the linkage is not blocked by other components. Manual controls may take up more panel space than other types of actuators.
- Air: These discharges use a pneumatic cylinder to open or shut the valve. The control is a simple switch that provides air to one side of the cylinder or the other. Pneumatic valves cannot be throttled.
- Electric: These discharges utilize an electric motor mounted on the valve body to open and close the valve. Operationally, these valves are the most flexible as the electric controls offer very clear displays of valve position, and also allow the operator to actuate the valve to a preset position at the touch of a button. These types of valves are equipped with a manual connection on the end of the actuator gear shaft that may be used to open or shut the valve in the event of a loss of electrical power. One advantage of using electric valves is the allowance of increased flexibility in apparatus design. Controls may be located without regard to where the valve is located, even if they are at opposite ends of the apparatus. Some designs allow controls in multiple locations. As a result, the plumbing may be designed to make the most effective use of the available space, thus allowing more space on the apparatus for other purposes. However, a disadvantage of these valve types may be that the upfront cost which are typically higher than manual actuators. However, the total cost (e.g. upfront versus warranty and maintenance) between electric actuators versus manual actuators should be considered.
DISCHARGE INSTRUMENTATION
Per NFPA® 1901 Standard for Automotive Fire Apparatus, certain fire apparatus under the standard require, all discharges 1.5”,or larger, to have an individual discharge gauge that visually indicates the hose inlet pressure immediately downstream of the valve. There is some variation allowed on other fire apparatus types not covered by the standard. (e.g. wildland fire apparatus)
There are many types of gauges that include, but are not limited to, simple coil-tube mechanical gauge and electronic transducers that provide a signal to a digital display. Regardless of gauge type, if the apparatus is to be operated in cold climates where ambient temperatures can fall below freezing for sustained periods of time, the gauges must be protected to prevent water from getting trapped in the gauge sensor and freezing. Freezing will damage the gauge. Digital pressure gauges are also available. These can be easily installed in the plumbing and the pressure can be displayed on the same controller that is used for operating the electric valve. This can simplify the pump panel design and layout.
Flow meters are becoming more common in the fire service. Flow meters use a mechanical or electronic device, usually a paddlewheel, to provide a visual indication of the flow rate through a discharge. This allows the operator to know exactly how much water is flowing at a given time. Electronic flowmeters can also allow cumulative measurement of all the water flowed through a given discharge over the course of a sustained period of time. If a mechanical and electronic flow meters are networked together, they can provide the total water flowed through all discharges that have a flow meter installed.
There are two potential disadvantages to flowmeters. First, they are often expensive relative to stand-alone pressure gauges. Second, they may require additional plumbing space since the inlet plumbing must be straight for a sufficient distance to guarantee smooth flow at the flowmeter so the flow measurements are accurate.
CHEMICAL INJECTION
Some discharges will require the introduction of firefighting chemicals such as foam concentrate. There are several methods to do this; from simple injection ports to full body eductors. The common feature of all methods is they will introduce additional flow restrictions into the discharge.
Direct injection foam systems introduce foam at a single point that feeds multiple discharges and mitigates additional restrictions in the flow. Therefore, this single injection point becomes the main flow restriction for all the discharges it feeds and must be sized to find the balance between injection accuracy and minimizing the flow restriction.
PLUMBING MATERIAL
The plumbing material should be designed for the agent that flows through it. Stainless steel is in common use and valves are typically made with a stainless steel or composite polymer balls and brass, or bronze, bodies. These materials provide excellent corrosion resistance for both foam and water. Steel piping is becoming rare and is usually only used to minimize expense.
Steel may be suitable for water even though the effects of corrosion may become evident much more quickly than for other materials. However, it should not be used for foam. Aluminum piping is not typically used for fire apparatus, except on waterways for aerial devices.
Regardless of the material, the plumbing system must be able to withstand the expected pressure rating of the pump with a sufficient safety margin (500 psi for normal pressure pumps, and higher for high pressure and ultra-high pressure pumps.)
HOSE AND APPLIANCE CONNECTIONS
The hose connections must be selected based upon the operational practices of the department. The most common connection on any discharge is a male National Standard Thread (NST). NST threads are required to allow full interoperability between departments in other regions.
Some jurisdictions specify other connections, such as Storz, for large diameter discharges. Some jurisdictions may still have custom threads on their hose based upon the history of their water system. If custom discharge threads are desired, it is required by NFPA 1901 that they be added in the form of adapters on the NST threads if the apparatus is to be used in the United States. This will maximize interoperability and allow the apparatus to be restored to the latest industry standards if it is ever sold.
Any discharge connection higher than 42 inches above the ground should be equipped with a turndown adapter to prevent kinking of the hose. All discharges should be equipped with a cap to protect the threads and prevent leakage when not in use. The caps should remain secured to the apparatus when removed from the connection using a restraining cable or chain.
Certain exceptions may, such as a deck gun or master stream device, that is designed to be mounted directly to the apparatus. Some models allow easy removal of the device so that it may be connected to a ground base supplied by one or more hose lines.
Purchasers of fire apparatus must confirm the connection type for deck guns. Deck gun manufacturers can supply their products with several different inlets from pipe thread to flanges, so it is important to make sure they match.
Drains should be supplied on any discharge larger than 1”. This will allow pressure to be drained from the hoseline regardless of what the pump is doing and without having to open the nozzle. Additional drains should be provided as necessary to allow full draining of all low points in the plumbing to provide protection against freezing.
Components
With any type of pump, there are several components that are common, and perform common functions. Some of these include:
- Various intake manifolds, piping and valves to control and direct water coming into the pump,
- Various discharge manifolds, piping, and valves to control and direct the water output
- Intake Pressure Relief Valves (PRV) to discharge excess pressure coming into the pump and protect the intake side of the pump and piping
- Discharge pressure relief valves and pressure governors to limit discharge pressures to protect discharge components and hose lines but also protect firefighters from undesirable pressure surges,
- Drain controls to drain water from the pump intake and discharge plumbing
- Pump control devices, throttle, governor, primer, pump cooler, engine cooler, drains, and thermal relief valves
- Pump monitoring devices and pump-engaged indicators, master intake and discharge pressure gauges, dedicated inlet and discharge pressure gauges, and flow meters (if so equipped)
- Engine monitoring devices such as engine speed, fuel level, oil pressure, cooling system temperature, electrical charging system
Foam system valves, controls, and monitoring devices (if so equipped)
Corrosion Prevention
Corrosion and material deposits can be an issue depending on the pump, plumbing, and valve materials utilized. The mineral and salt content of the water source can also accelerate corrosion or build-up of deposits. A sacrificial anode helps reduce galvanic corrosion within the pump body by “attracting” the corrosion away from the main parts of the pump. In simple terms, galvanic corrosion is an electric circuit, like a battery. The anode is more active electrically than the pump components, and therefore provides the electric flow that attracts corrosive material away from the parts you want to protect. Because it is sacrificial, the anode will experience decay and will have to be replaced periodically. If the anode is not replaced, once it is depleted, corrosion will likely attack pump components.
Anodes do not provide protection against other forms of corrosion such as general oxidation, stress corrosion, etc. All pumps, valves, appliances, and related components must be tested at least annually in order to assure continued safe and reliable operation.
Annual Pump and Related Equipment Testing
The pumps and associated equipment should be tested annual per the NFPA 1911 Standard for the Inspection, Maintenance, Testing, and Retirement of In-Service Automotive Fire Apparatus. Test procedures for external valves and appliances can be found in the NFPA 1962 Standard for the Care, Use, Inspection, Service Testing, and Replacement of Fire Hose, Couplings, Nozzles, and Fire Hose Appliances.
Often a thorough Preventative Maintenance Program will detect issues early before they become major, expensive problems that may take the apparatus out of service.
Appendix A – Abbreviations
Appendix A – Abbreviations click here to download chart.
Appendix B – Pump Drive Mounting Diagrams
Appendix C – Manufacturer Contacts
For further information on apparatus and component manufacturers, refer to the FAMA Members List.